This paper examines the research, design principles, and production techniques for anchor flanges, which are vital support components in large-scale gas and oil pipelines operating under high pressure and spanning long distances. By prioritizing advanced and practical design principles, domestically produced anchor flanges now meet the rigorous requirements of major infrastructure projects.
Anchor flanges are critical support components for large-diameter, high-pressure, long-distance gas and oil pipelines. These pipelines operate under demanding conditions, exposed to complex forces and stringent technical requirements, which create significant manufacturing challenges. Transporting energy from remote oil and gas fields to industrial centers is a key part of China's energy policy, driving economic development and improving living standards. For example, the West-East Gas Transmission Project, launched in the early 2000s, delivers natural gas from the Tarim Oil and Gas Field in Xinjiang to coastal regions. This 4,000-kilometer pipeline spans 10 provinces, crossing diverse terrains, including the Gobi Desert, Huangshi Plateau, Taihang Mountains, and major rivers such as the Yellow, Yangtze, and Huai. It faces seasonal climate extremes, complex geological conditions, and challenges from corrosion and vibration, requiring exceptionally reliable pipeline components.
Previously,
anchor flanges were predominantly imported, limiting project development. To accelerate energy infrastructure progress, China has undertaken extensive scientific research and localization efforts. Recent breakthroughs in independent innovation have enabled the development and production of anchor flanges that meet the rigorous demands of major projects. In the coming years, new gas and oil transmission projects, such as the "Sichuan Gas Out of Sichuan" and the "West-East Gas Transmission Second Pipeline," will be launched alongside increasing oil and gas imports. Therefore, China's manufacturing sector must prioritize the innovation of advanced products and cutting-edge manufacturing technologies.
The anchor flange is welded to the main transmission pipeline and anchored with cement piers (as shown in Figure 1) to mitigate excessive pipeline displacement caused by combined forces, ensuring the safe operation of the pipeline and booster pump station. The primary forces acting on the anchor flange include internal pipeline pressure and the resulting axial force (Poisson's force), thermal stress due to temperature fluctuations, axial forces resulting from changes in pipeline direction and orientation, and additional torque from the pipeline’s weight and thermal expansion or contraction.
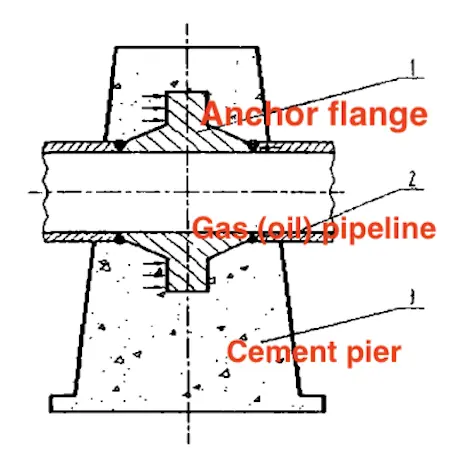
Figure 1 Working conditions of the anchor flange
Underground pipelines face uneven soil and water pressure, while above-ground sections are exposed to environmental forces such as wind, snow, and seismic loads. Due to the complexity of these forces, it is critical to evaluate the flange’s strength, stiffness, fatigue resistance, brittle fracture resistance, and seismic performance. Certain stresses are challenging to quantify accurately, prompting ongoing global research in this area. In the engineering design, the pressure vessel codes are followed, relevant standards and practical data are referenced to ensure an increased safety margin. Engineering dimensions are determined through finite element analysis and structural design.
In compliance with international standards (ASME), pressure vessel regulations (China’s national standards), and industry-specific technical specifications, the design stress coefficient (0.4) is calculated according to the actual production conditions of materials. The flange outer diameter (D) is determined with the total axial thrust (equivalent force) and the compressive strength of the cement pier (10.3MPa). The flange thickness (L) is derived from the bending moment acting on the flange, while the port structure dimensions are determined according to welding specifications.
To analyze the stress distribution of the anchor flange during operation, the latest MSC.MARC software is used to model the equivalent axial thrust, temperature fluctuations, and other specified factors. This analysis generates a stress distribution map, indicating the size and location of stress concentrations (see Figure 2). Finally, stress is simplified, and components are categorized and verified to ensure the system operates safely and reliably.
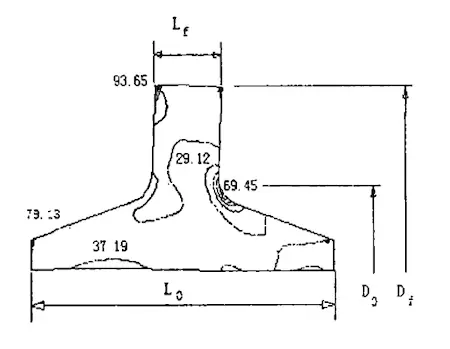
Figure 2 Finite Element Stress Analysis and Structural Design
Material selection focuses on key properties such as high mechanical strength, processability, and weldability. Additionally, materials must be innovative, suited to conditions in China, and easy to manufacture. For pipeline steel, API 5L X70, developed by the American Petroleum Institute, is selected. This grade provides exceptional resistance to dynamic and static loads, superior durability against oil and gas erosion, and excellent performance in low temperatures, including resistance to hydrogen-induced cracking and sulfide stress corrosion.
Plastic forming and heat treatment are essential for ensuring structural integrity, optimizing streamline distribution, and refining uniform grain structures. Moreover, the hot working process improves material efficiency, energy savings, defect reduction, and cost-effectiveness. Given the current capabilities of hot working equipment, an optimized process plan has been developed.
When the capacity of the ring rolling machine is insufficient, rotary forging and hole expansion processes serve as effective alternatives. The key factor in these processes is maintaining precise control over the deformation temperature range and ensuring uniform rotation during each forging step. Adequate deformation, combined with well-regulated thermal parameters, guarantees high-quality forging results. To maximize material efficiency, the flange edge should be forged outward. The process may use an upper grooved anvil for the mandrel hole expansion or place a pre-expanded blank flat on the lower anvil, with a rotating device employed for simultaneous forging and punching.
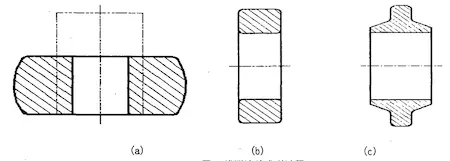
(a) Upsetting Punching (b) Pre-expansion (c) Final Forging
Figure 3 Anchor Flange Forming Process
Practical experience has shown that precise control of deformation temperature, speed, and degree, along with evenly distributed strain, consistently produces products that meet quality standards. This method represents the optimal forming approach.
Controlled ring rolling, similar to advanced controlled rolling and cooling technologies, is performed on humanoid ring rolling machines by precisely managing thermodynamic parameters. This process produces products with superior intrinsic quality, high production efficiency, minimal processing allowances, and suitability for mass production.
Cast ring billets, or billets produced by medium-frequency melting or electro-calcining, are briefly heated uniformly before being rolled or forged into shape. This process is energy-efficient (with reduced heat consumption) and involves fewer steps, minimizing material waste and reducing production costs. By precisely controlling the hot forming parameters of the ingot, high-quality, efficient, and cost-effective production is achieved.
In summary, controlled hot forming and streamlined processes are advanced manufacturing methods that warrant further development. Large ring components often exhibit shape and size variations due to various factors. These variations must be corrected before machining to minimize processing allowances. Shaping devices may include mechanical wedge-expansion systems, diameter adjustment tools, or hydraulic expansion shaping equipment.
To meet technical specifications, the mechanical properties of the workpiece must be optimized. After forming, the anchor flange undergoes tempering. Electric furnace temperature control is the preferred heat treatment method; however, to conserve electricity in constrained situations, preheating in a gas furnace followed by electric furnace processing can maintain quality while reducing energy consumption. Post-forging, the anchor flange undergoes multiple quality inspections and a water pressure test. Given the large diameter of the workpiece and high sealing force requirements, efficiency-enhancing devices can conduct water bed tests on small-tonnage presses. These technologies are widely applicable in production environments.
The production of large castings and forgings is crucial to the manufacture of critical equipment. To advance production localization and modernize the equipment manufacturing industry in China, it is essential to focus on independent innovation, research, and design while addressing key technological challenges. Developing advanced and practical technologies, delivering high-quality products, meeting urgent project needs, and contributing to social and economic progress are key priorities.